An introduction to therapeutic proteins
Table of contents
ShowTherapeutic proteins have become indispensable tools for treating a wide range of diseases and medical conditions. These biologically derived molecules offer targeted solutions that can provide significant benefits over traditional pharmaceuticals. From monoclonal antibodies to enzymes, hormones to growth factors, therapeutic proteins encompass a diverse array of molecules with distinct roles in healthcare.
This article provides an overview of therapeutic proteins, exploring their various types, applications, challenges, and recent advancements. Also, we will discuss the biopharmaceutical processes and advancements in the production of therapeutic proteins.
Therapeutic proteins – an overview
Therapeutic proteins are biologically derived molecules that are utilized for medicinal purposes to prevent, treat, or cure diseases and medical conditions. These proteins are typically produced through biotechnological methods, often using recombinant DNA technology, to create precise and targeted treatments.
Therapeutic proteins can take various forms, including antibodies, enzymes, hormones, growth factors, cytokines, and fusion proteins. They are designed to interact with specific molecular targets in the body, such as receptors, enzymes, or signaling molecules, to modulate biological processes or restore physiological functions.
The development of therapeutic proteins has significantly expanded the scope of medical interventions, offering tailored treatments for a wide range of conditions, including cancer, autoimmune diseases, metabolic disorders, infectious diseases, and genetic disorders. They provide advantages over traditional small molecule drugs by offering reduced side effects, enhanced specificity, and the potential to perform complex tasks.1
Types of therapeutic proteins
Characterizing them according to their molecular types, the following classes of therapeutic proteins can be distinguished (three of which shall be further explained below):
Antibody-based drugs
- Designed to target specific molecules in the body
- Used in cancer, autoimmune diseases, and inflammatory disorders
Fc fusion proteins
- Combine the Fc region of an antibody with another protein or peptide
- Enhance stability and half-life, used in cytokine therapy and drug delivery
Anticoagulants
- Interfere with blood clotting to prevent thrombotic disorders
- Examples include heparins and thrombin inhibitors
Blood factors
- Involved in the blood clotting cascade
- Treat bleeding disorders like hemophilia
Bone morphogenetic proteins (BMPs)
- Stimulate bone formation and repair
- Used in orthopedic surgeries for bone healing
Engineered protein scaffolds
- Mimic natural proteins for drug delivery and tissue engineering
- Versatile platforms in drug development
Enzymes
- Replace or augment deficient enzymes
- Treat enzyme deficiencies and metabolic disorders
Growth factors
- Regulate cell growth and tissue repair
- Stimulate tissue regeneration in various conditions
Hormones
- Regulate physiological processes
- Used in hormone-related disorders like diabetes
Interferons
- Activate immune response to viral infections and cancer
- Treat hepatitis and certain cancers
Interleukins
- Regulate immune responses and inflammation
- Used in cancer and autoimmune disease treatment
Thrombolytics
- Dissolve blood clots to treat thrombotic events
- Used in myocardial infarction and stroke treatment
Source: NCBI2
Antibodies and ADCs
Antibodies, along with B-cells, T-cells, and phage cells, represent a vital component of the immune system, recognizing and neutralizing foreign pathogens. Yet, they can also be engineered in order to modulate a patient’s immune response in a controlled manner. Among them, monoclonal antibodies (mAbs) are engineered to specifically target antigens present on diseased cells or pathogens, acting as inhibitors, inducers of immune responses, or carriers of cytotoxic payloads to target cells.
Promising in this context is the development of antibody-drug conjugates (ADCs), a type of targeted therapy amalgamating antibody specificity with the cytotoxic potential of chemotherapy drugs. ADCs consist of an API conjugated to a monoclonal antibody, facilitating precise drug delivery to cancer cells expressing the target antigen. Upon binding, the ADC is internalized, releasing the cytotoxic payload and inducing cell death.
This advancement addresses challenges in chemotherapy, mitigating off-target effects and systemic toxicity. ADCs offer promise in overcoming drug resistance and treating cancers with limited therapeutic options. As research progresses, optimizing ADC design and delivery methods continues, fostering hope for patients with cancer and other complex diseases.
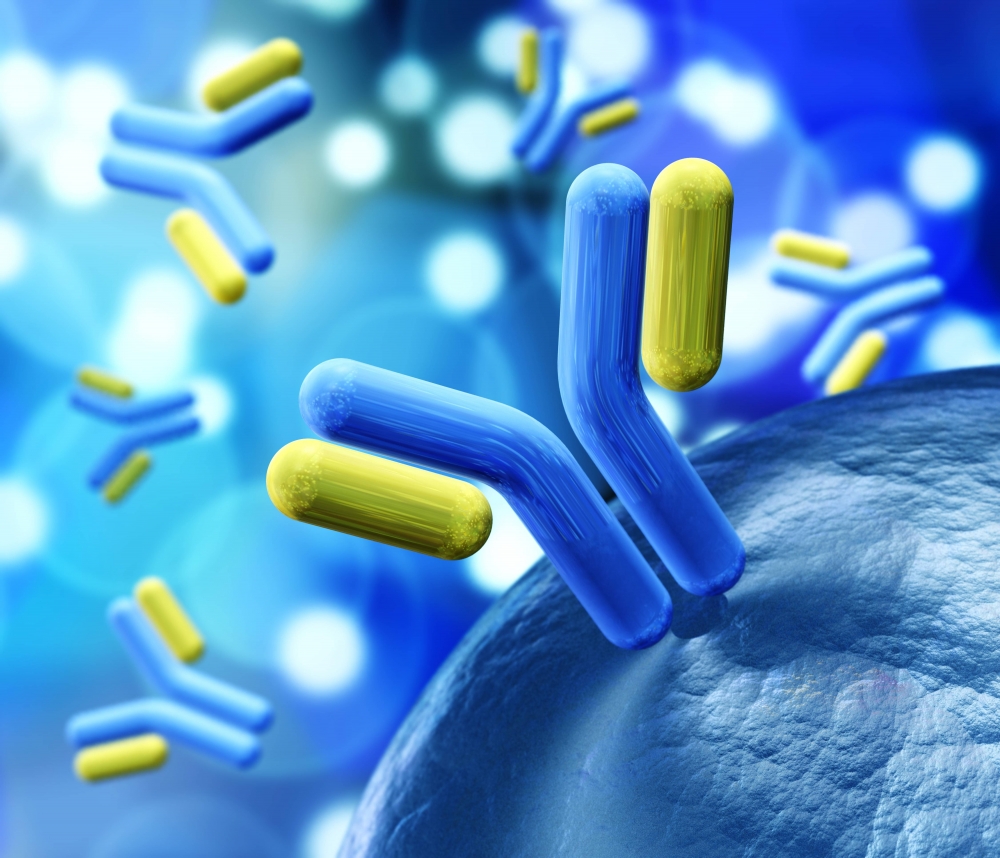
Enzymes and hormones
Enzymes and hormones, both not only occurring naturally, but also as therapeutic proteins, offer targeted treatments for various medical conditions.
Enzymes catalyze biochemical reactions in the body; recombinant enzymes are used to manage enzyme deficiencies and metabolic disorders.
Hormones, on the other hand, regulate physiological processes. Recombinant hormones treat hormone-related disorders such as diabetes and growth disorders.
Examples and applications of protein therapeutics
Protein therapeutics, including peptides and recombinant proteins, are a valuable addition to the treatment portfolio in modern medicine. Designed for in vivo use, these molecules exhibit favorable pharmacokinetics and find diverse applications.
They are crucial components in vaccines, driving immune responses against infectious diseases. Additionally, protein drugs, such as monoclonal antibodies and fusion proteins, target specific therapeutic targets in biopharmaceuticals (e.g. the chimeric IgG Rituximab for cancer treatment)3. Moreover, protein-based drug delivery systems efficiently transport small molecules to therapeutic targets.
The substitution of human proteins like enzymes or hormones via analogues is another substantial application of therapeutic proteins: Examples include Novo Nordisk’s modified insulin or Roche’s human EPO.3
Challenges and chances with protein therapeutics
In the context of therapeutic proteins, challenges and opportunities go hand in hand. In terms of development and application, one of the main challenges is to mitigate unwanted immune reactions due to high immunogenicity.4
Different regulations by authorities like the FDA in the USA or the EMA in the EU pose hurdles for developers, while comprehensive characterization and toxicity management remain essential tasks. Protein aggregation during production and storage presents a risk, as does the complexity of polymer-based delivery systems. The overall intricate manufacturing process of therapeutic proteins – from formulation to transport – requires strict compliance, high-performing fluid and cold chain management solutions, and elevated safety levels.
Despite these challenges, recent advances in protein engineering offer promising chances for optimization. Immunomodulation potentializes therapeutic proteins for treating autoimmune diseases, while novel ADCs hold great promise in the fight against cancer. In addition, other severe diseases, like multiple sclerosis or anemia, can be treated with therapeutic proteins as well. And with several approaches currently in preclinical development or clinical trials, along with the multiple already FDA-approved products, the success story of therapeutic proteins is far from being over.4
Advancements in therapeutic protein development
Advancements in therapeutic protein development have propelled the field forward, revolutionizing treatment options for various medical conditions. Through innovative in vitro techniques and technologies, such as PEGylation, albumin-fusion, amino acid manipulation and glycosylation strategies, researchers can optimize the properties (e.g. molecular weight) and functionality of therapeutic proteins. Innovations include fusion proteins, combining therapeutic properties.5
Recombinant DNA technology enables efficient production in various expression systems, while methodological innovations streamline the purification and characterization processes. Open access initiatives foster collaboration and knowledge sharing, accelerating progress in therapeutic protein research.
Production process of therapeutic proteins
As already discusses, the variety of different therapeutic proteins is huge; accordingly, the production methods differ as well. Recombinant DNA technology, cell culturing, genetic manipulations and the generation of polypeptides with complex post-translational modifications – this is only a small excerpt from what therapeutic protein production may involve.
Apart from specialized approaches, there are indeed some overlapping similarities – process steps that many therapeutic proteins have in common, as highlighted in the following chapters.
Aseptic fluid management of therapeutic proteins
The production process of therapeutic proteins often involves the use of mammalian cell expression systems. A crucial aspect of this process is aseptic fluid management, ensuring the sterility and integrity of the product. This methodology is essential for maintaining the quality and efficacy of therapeutic proteins, including biosimilars, throughout their production.
But not only during cell culturing, aseptic fluid management is essential: The entire fluid path needs to be protected from contamination, disruptions, and physical stress that can have an impact on product quality. Considering the changing volume sizes – therapeutic proteins are produced not only in bulk, but also on a smaller scale –, modular and closed processes are being increasingly adopted to provide a protected production environment.5
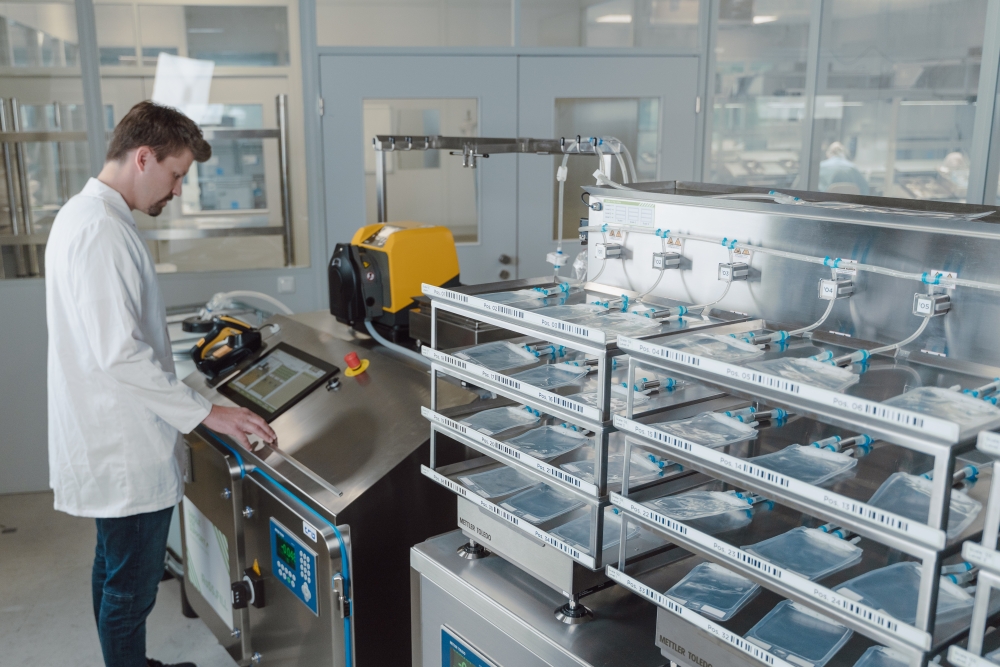
Therapeutic protein freezing – how to maintain stability
In order to maintain protein stability, adequate freezing methods have to be individualized and established.
For instance, controlling the freezing rate is crucial. Uncontrolled freezing rates may lead to the formation of large ice crystals, causing mechanical stress and potential denaturation of proteins. In contrast, controlled freezing, such as plate freezing, minimizes the formation of ice crystals and preserves protein structure more effectively.
Plate freezing offers distinct advantages over uncontrolled slow freezing in maintaining protein stability. With plate freezing, proteins come into direct contact with the cooled plates, facilitating rapid and uniform freezing. This direct contact minimizes the risk of ice crystal formation and ensures consistent cooling throughout the protein solution. In contrast, static freezing relies on cold air circulation, which may result in uneven cooling and the formation of larger ice crystals, potentially compromising protein stability.
Furthermore, adequate protein storage conditions are paramount for preserving protein stability post-freezing. This not only includes selecting an appropriate storage temperature, but also to protect the proteins from temperature fluctuations and exposure to light. Protective packaging and storage in a controlled environment help mitigate the risk of temperature fluctuations and ensure the integrity of the frozen therapeutic proteins.
Optimization of therapeutic protein production with single-use technologies
Optimization of therapeutic protein production is vital for ensuring efficient and cost-effective manufacturing processes. Single-use technologies offered by Single Use Support play a crucial role in enhancing various aspects of therapeutic protein production, including filing, freezing, and storage.
In terms of aseptic aliquotation, single-use technologies enable streamlined and flexible processes. These technologies eliminate the need for time-consuming and costly cleaning and sterilization procedures associated with traditional stainless steel equipment. Instead, single-use systems, such as the RoSS.FILL filling system, offer fully automated and disposable solutions for filling therapeutic proteins into single-use bags. This simplifies the dispensing process, reduces the risk of contamination, and allows for rapid product changeovers, thus optimizing production efficiency.
When it comes to freezing, single-use technologies offer advanced solutions for maintaining the stability of therapeutic proteins. The RoSS.pFTU freeze-thaw platform provides precise control over the freezing process, minimizing the formation of ice crystals and preserving protein structure. This is crucial for preserving the efficacy of recombinant proteins and other biologics during freezing and storage.
As of storage, single-use technologies offer flexible and scalable solutions for maintaining the integrity and safety of therapeutic proteins. The RoSS® Shell provides a robust and secure transport solution for all available single-use bioprocess containers, ensuring safe storage and transportation of drug substances. Furthermore, Single Use Support's ultra-cold storage freezer, the RoSS.ULTF, offers precise temperature control down to -75°C, ideal for preserving the stability of sensitive biologics for diagnostics and therapeutics.
Single-use technologies provided by Single Use Support offer significant advantages in optimizing therapeutic protein production. From streamlined filing processes to precise freezing and reliable storage solutions, these technologies enhance efficiency, minimize contamination risks, and ensure the integrity and stability of valuable biopharmaceutical products throughout the production lifecycle.
- Therapeutic Protein, https://www.sciencedirect.com/topics/biochemistry-genetics-and-molecular-biology/therapeutic-protein, Published 2014
- Therapeutic Proteins, http://dx.doi.org/10.1007/978-1-61779-921-1_1, Published 2012-11-30
- Therapeutic Proteins, http://dx.doi.org/10.1007/978-1-61779-921-1_1, Published 2012-11-30
- Immunogenicity of Protein-based Therapeutics, https://www.fda.gov/vaccines-blood-biologics/biologics-research-projects/immunogenicity-protein-based-therapeutics , Published 03/2024
- Recent advances in (therapeutic protein) drug development, http://dx.doi.org/10.12688/f1000research.9970.1, Published 2017-02-07