Stressing Cells: The Role of Cryoprotectants in ATMP Cryopreservation
Table of contents
ShowIn cell and tissue therapies, living cells are used to treat previously chronic diseases, like cancer forms, arthritis, or certain autoimmune disorders. To be effective, the living therapeutic product requires administration with a sufficient cell number at a high cell viability and functionality. Freezing of cells during cryopreservation attempts to target those quality requirements by simultaneously ensuring a prolonged shelf life of the starting material as well as the final therapeutic product. This provides greater flexibility for patients and during the manufacturing process. Cryopreservation accompanies the entire manufacturing and logistics process of Advanced therapy medicinal products (ATMPs) and thus has an immense impact on the therapeutic success and safety of the product1.
Cells and tissue therapies that are currently approved, originate from a variety of cell types like dendritic cells, chimeric antigen receptor T (CAR-T) cells, haematopoietic stem cells, fibroblasts, chondrocytes, limbal stem cells and adipose-derived stem cells1. Due to the variety of cell types as starting material and their different optimal conditions during bio-cryopreservation, each cell and tissue therapeutical product requires its own specific and coordinated cryopreservation workflow to maintain the viable recovery and therapeutical efficacy2.
Fluid and Cold Chain Management of ATMPs
Unlike centralized manufacturing of large identical batches, autologous ATMPs are patient-specific with one batch of cell product, and distant manufacturing sites3 making them incompatible with typical supply chains. Starting material and the final product forming a cryogenic cold chain. This chain ensures the integrity of materials during transportation, storage, and thawing, completing the autologous ATMP supply chain. The process involves patient assessments, cell collection, cryopreservation, transportation to the manufacturing site, manufacturing and manipulation, transportation, and final thawing at the clinical site, before final administration2.
Cryopreservation offers scheduling flexibility, minimizes logistical risks, and allows for timing that best suits the patient. Additionally, it provides benefits such as accommodating delays and eliminating time constraints for initiating manufacturing. All that mentioned therapeutic advantages can be attributed to an increased shelf life of the therapeutic product4.
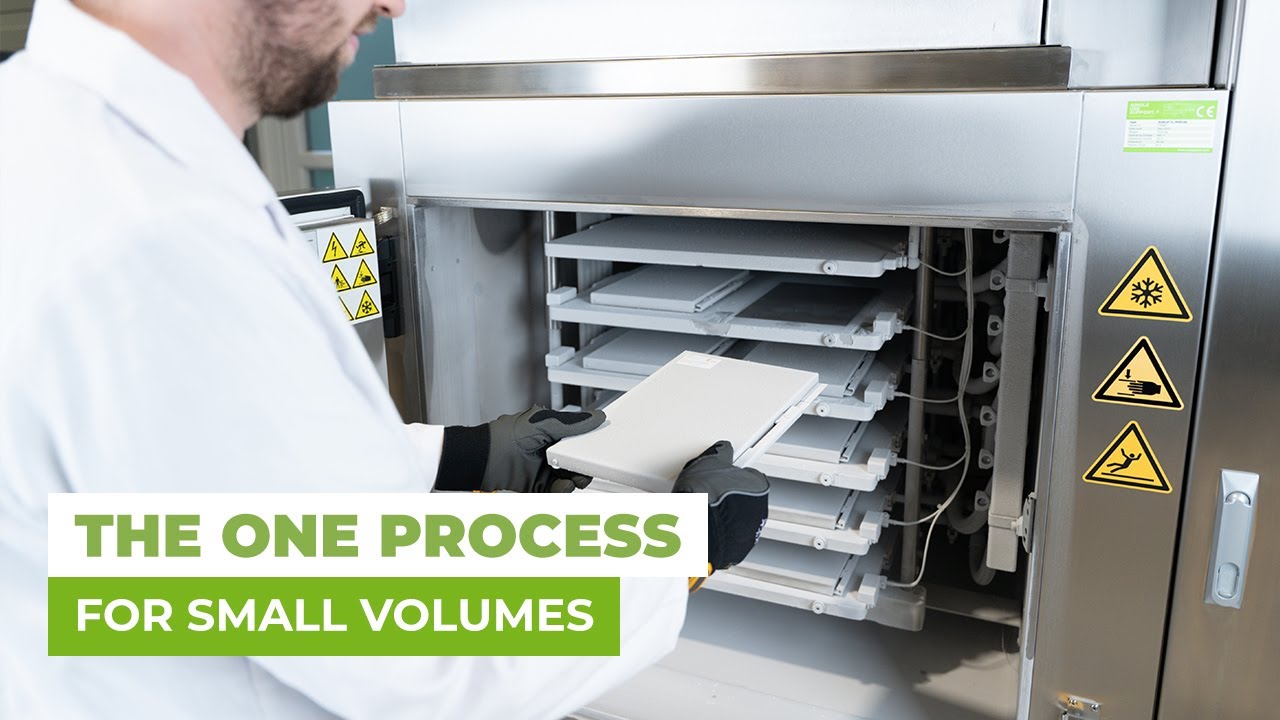
Increasing ATMPs shelf life
At the very early stage in the ATMPs supply chain, as soon as cells are removed from their natural environment, they start to lose function. Keeping somatic cells outside the body viable and functional active for an extended period can be carried out by different approaches. One strategy is to mimic the cells natural condition within a controlled aseptic system to enable all cell relevant functions (metabolism, expression, signalling & transport). Most cell types (except cancer cells) can be ex-vivo cultured for a limited number of passages until the cells loses proliferative potential and accumulate mutations.
Keeping Cells Alive Outside Their Comfort Zone
Cryopreservation follows an opposite approach and attempts to shut down metabolic activity completely, at temperatures below -130°C. Compared to cells undergoing cryopreservation, cells in the cell culturing setting live a quite comfy life; with steady temperatures at around 37°C degree, sufficient oxygen, continuous fresh media, and even their waste gets removed. On the opposite, cryopreserved cells must deal with extreme conditions like ice crystals that are going to punctuate their cell membrane, severe dehydration due to freezing induced osmosis, and cell toxic cryoprotectants (especially at ambient temperature).
Exposing cells to such conditions might seem counterintuitive on the first glance, thereby cryopreservation must be seen under the light of arresting biological degradation and conserving cellular functionality by immobilizing water through freezing. The objective of an optimal cryopreservation strategy is to deactivate (put on hold) degenerative cellular pathways and preserve proliferative potential by reducing the temperature below –130°C, this halts molecular transport, allowing cells to enter a state of "suspended animation" without compromising the quantity, quality, viability, and recovery of cells2.
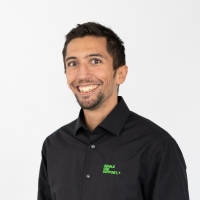
Cryopreservation must be seen under the light of arresting biological degradation and conserving cellular functionality by immobilizing water through freezing.
Khalil EssaniBiophysics of freezing cell therapeutics
In order to understand cryopreservation and the role of cryoprotectants, it is necessary to look at the biophysical behaviour of water in a cellular context.
Water molecules have a dipole nature due to their OH-group, that is engaged in braking and reforming of weak bonds. As water is cooled, molecules get closer to each other, and the breaking of bonds diminishes. Upon reaching a critical point in local thermal conditions, known as the phase transition, hydrogen and oxygen molecules start forming sufficient H-bonds (4 hydrogen bonds), reorganize in a more space demanding hexagonal structure and initiating the creation of an 'ice embryo' through a process known as ice nucleation5.
The formed ice crystals lead to a reduction in the free water available for cellular processes. Water molecules that normally are involved in the solvation of (salts, proteins, lipids, sugars) molecules, getting removed from the hydration shells of the solute molecules, while joining the formation of ice crystals. Consequently, the solutes within the solution become more concentrated as water is locked in the ice lattice. This results in water efflux (transport out of the cell) to establish osmotic equilibrium within the cell. Especially during slow freezing more time for water removal out of the cell is provided, resulting in increased cell dehydration (cell shrinkage). During cryopreservation, the drop in temperature typically induces extracellular ice formation, except in cases of rapid freezing. In a rapid freeze protocol, a cell struggles to establish equilibrium with the external environment due to the rapid formation of extracellular ice, which limits the continuous water transport from inside the cell to the extracellular environment. Consequently, the cytoplasm becomes increasingly super-cooled (higher solute concentration, decreases freezing point), elevating the likelihood of nucleation and subsequent intracellular ice formation5.
An effective cryopreservation strategy aims to prevent intracellular ice formation during the transition from the aqueous phase to the ice phase. Success in cryopreservation hinges on achieving the glass transition temperature (-123°C), wherein the liquid transforms into a solid state, without compromising the quantity, quality, viability, and recovery of cells.
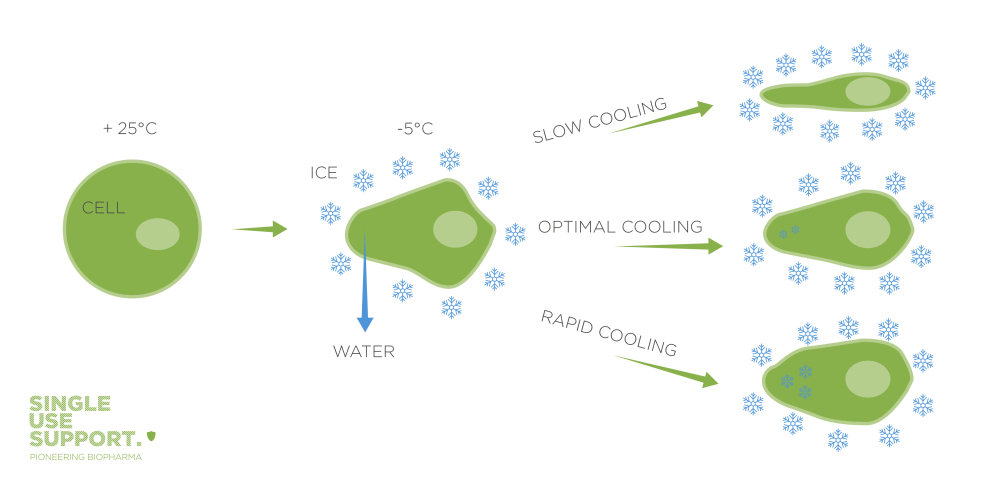
The role of cryoprotectants in cell freezing
Cryopreservation has the potential to induce various cellular injuries (f.e freezing induced increase in osmolarity, physical punctuation), which may result in adverse alterations in cell morphology, characteristics, proliferation ability, and function. To reduce the effects of ice crystal injuries, and osmotic injuries, cryopreservation protocols usually incorporate cryoprotective agents (CPAs). These agents operate through diverse mechanisms, such as lowering electrolyte concentration and hindering ice formation by forming hydrogen bonds with water molecules, thereby preventing their association with ice crystals.
The general chemical structure of a CPA contains a polar group, by which it interacts with the water molecules through forming hydrogen bonds. The hydrogen bonds between the cryoprotective agent and the water molecule are stronger than the water-water molecule interaction (hydrogen bonds). In other words, the cryoprotective agent attracts water molecules and thereby removes free water molecules that otherwise would be accessible to form tight water-water molecule interaction, that are needed for critical sites for crystal nucleation6.
Read more: Evaluating freeze-thaw processes in antibody production
Permeating agents vs. Non-permeating agents
Permeating cryopreservation agents are highly water soluble at low temperatures, contain a polar group that facilitates interaction with the OH-group of water, are of amphiphilic and have a small size (100 da) to diffuse through the cell membrane. The permeating feature enables the CPA to remove water from the intercellular space, and thereby supressing the ice formation inside the cell. Furthermore, a good permeating cryoprotectant, should balance osmotic imbalances and should be low toxic to the cell. The most common permeating CPA include, DMSO, ethylene glycol, propanediol, glycerol. All of them are of small size and contain a hydrophilic as well as hydrophobic feature (giving them an amphiphilic property) that enables easy transport along the amphiphilic cell membrane6.
Non-permeating CPA are macro molecular cryoprotectants that include sugars, polymers, and proteins which are excluded from transportation across the cell-membrane, either due to their large size or their polarity nature and the lack of an adequate transport system7. There function is also based on attraction of water through highly polar groups. They can be dimers, trimers, or polymers. Some common non-permeating CPA are polyethylenglycocol (PEG), polyvinylpyrrolidone, raffinose, sucrose, trehalose. There are approaches, that for non-permeating agents, like trehalose, the cell is genetically modified to express a trehalose transporter to allow transport across the membrane.
Challenges in Adding Cryoprotectants
Cryoprotectants are critical in facilitating successful cryopreservation techniques. Nevertheless, they impose two principal risks for biological samples:
- Cytotoxicity and
- osmotic shock associated with their introduction or removal
Non-penetrating CPA are usually less toxic than penetrating CPA, but also less effective. In practice, usually a combination of both is applied, to control water efflux and attract water molecules. Though penetrating CPA are quite small, they still require a certain time to diffuse inside the cell and establish a chemical equilibrium. To allow sufficient internalization of intracellular CPA, the cells are typically incubated with the CPA for a couple of minutes, depending on the specific cell type. Notably, in the case of dimethyl sulfoxide (DMSO), cytotoxicity exhibits a temperature-dependent behaviour, necessitating its incremental addition to a pre-chilled sample7.
The setup of a cooling and homogenizing cells prior to aliquoting and freezing helps to extend the window to prevent damages to cells. RoSS.PADL and RoSS.FILL CGT are such examples where cells are cooled and kneaded simultaneously to facilitate a cell aliquotation with consistent cell counts from bag to bag (see image below).
Furthermore, the time between addition of cryoprotectant and cryopreservation should be minimised to limit any cytotoxic effects, but long enough to allow CPA internalization. The same has to be considered during thawing, in which the contact time between the CPA and the cells should be reduced during ambient temperature to a minimum. Following cryopreservation, apoptosis and necrosis typically manifest within 6 to 24 hours during post-thaw culture. This leads to a substantial decline in cell viability and compromised cellular function due to the cryopreservation process. The dysregulation of biochemical pathways after cryopreservation varies among different cell types, underscoring the importance of adopting a "cell-type dependent" approach. Existing literature indicates that T-cells, for instance, experience apoptosis after cryopreservation, with approximately 40% of cells undergoing apoptosis 8 hours post-thaw, followed by extensive cell death8.
Enhancing ATMP Cryopreservation - An Outlook
The past has shown that there is the continuous attempt for updating and harmonization of guidelines and standards. In the future a focus on stability studies to determine the shelf life in an adequate and meaningful way will be introduced and aligned. Such actions can significantly reduce the cost of stability studies without compromising safety as well as to support the comparison of data from manufacturing and especially cryopreservation.
From a material aspect, attempts to substitute cell toxic CPA - produced under GMP conditions and approved for therapy - with less toxic ones or certain mixes are under investigation. Less toxic CPA would allow to increase their concentration, and subsequently enables to apply a faster freezing scenario.
Implementation of safe integrated block chain solutions within the supply chain, in combination with Industry 4.0 and the Internet of Things, will enable a fully and detailed tracking - from verification of the origin and quality of materials to the unique batch-record of the therapeutic product inside the administered cryo-bag and its bag-history (temperature records). Furthermore, it enables the interoperability between different stakeholders in the supply chain, such as single-use equipment supplier, filling and freezing unit supplier, manufacturer, transport company, clinical centre. This allows seamless data sharing and collaboration while maintaining data security. This will secure therapeutic safety and efficacy but also eases to fulfil the regulations regarding record-keeping and documentation.
The overall challenge in cryopreservation for ATMP is the dependence on manual processes during the research phase and its transition to a commercial, automated, closed cryopreservation workflow, where entire processes need to occur within very tight parameter limits. These would be filling time, incubation time and temperature for CPA incubation, freezing rate inside the bag.
References
- Iglesias-Lopez C., et al.: Current landscape of clinical development and approval of advanced therapies, DOI: 10.1016/j.omtm.2021.11.003. Available at: Current landscape of clinical development and approval of advanced therapies - PubMed (nih.gov)
- Hawkins, B, et al: Biopreservation Best Practices for regenerative medicine GMP manufacturing & focus on optimized biopreservation media. Cell Gene Therapy Insights 2017; 3(5), 345-358. doi: 10.18609/cgti.2017.035Available at: insights.bio/cell-and-gene-therapy-insights/journal/article/410/Biopreservation-Best-Practices-for-regenerative-medicine-GMP-manufacturing-focus-on-optimized-biopreservation-media
- Jandova M. et al.: The role of cryopreservation techniques in manufacturing, transport, and storage of Car-T therapy products. Cryo Letters. 2023 May-Jun;44(3):123-133. Available at: https://pubmed.ncbi.nlm.nih.gov/37883165/
- Coopman, K., Medcalf, N.: From production to patient: challenges and approaches for delivering cell therapies. In: StemBook [Internet]. Cambridge (MA): Harvard Stem Cell Institute; 2008. 2014 Mar 31. Available at: https://pubmed.ncbi.nlm.nih.gov/24945057/
- Murray, K., Gibson, M.: Chemical approaches to cryopreservation. Nat Rev Chem. 2022;6(8):579-593. doi: 10.1038/s41570-022-00407-4. Epub 2022 Jul 18. Available at: https://pubmed.ncbi.nlm.nih.gov/35875681/
- Whaley, D. et al.: Cryopreservation: An Overview of Principles and Cell-Specific Considerations, Chemical approaches to cryopreservation, Cell Transplant. 2021 Jan-Dec; 30: 0963689721999617. Available at: https://www.ncbi.nlm.nih.gov/pmc/articles/PMC7995302/
- Murray, K., Gibson, M.: Post-Thaw Culture and Measurement of Total Cell Recovery Is Crucial in the Evaluation of New Macromolecular Cryoprotectants, DOI:10.1021/acs.biomac.0c00591, Available at: https://www.researchgate.net/publication/341957118_Post-Thaw_Culture_and_Measurement_of_Total_Cell_Recovery_Is_Crucial_in_the_Evaluation_of_New_Macromolecular_Cryoprotectants
- Sarkar S. et al.: Caspase-mediated apoptosis and cell death of rhesus macaque CD4+ T-cells due to cryopreservation of peripheral blood mononuclear cells can be rescued by cytokine treatment after thawing. Cryobiology. 2003;47:44–58. doi: 10.1016/S0011-2240(03)00068-3. Available at: https://pubmed.ncbi.nlm.nih.gov/12963412/