Non-viral vectors in gene therapy: LNPs in the spotlight
Table of contents
ShowGene therapy, a groundbreaking approach in modern medicine, holds immense promise for the treatment of various genetic diseases and cancer types. Central to the success of gene therapy are the vectors used to deliver therapeutic genes like mRNA and other nucleic acids into target cells.
While viral vectors have traditionally dominated the field, non-viral vectors have emerged as an alternative with significant advantages. Non-viral vectors, such as lipid nanoparticles (LNPs), offer a safer and more versatile means of delivering genetic material in both in vitro and in vivo settings. By circumventing an unwanted immune response associated with viral vectors, non-viral gene therapy opens up new avenues for clinical applications, particularly in cancer therapy.
Understanding non-viral vectors
Gene therapy has emerged as a promising approach for treating genetic diseases by introducing therapeutic nucleic acids into target cells. Non-viral vectors play a crucial role in facilitating the delivery of these genetic materials. Unlike viral vectors, which have limitations such as immunogenicity and limited cargo capacity, non-viral vectors offer advantages in terms of safety, versatility, and ease of synthesis and formulation. Furthermore, they are easier to manufacture, but also to scale.
Types of non-viral vectors
- Polymer-based non-viral vectors
One type of non-viral vectors are polymers. Including synthetic and natural polymers, they have gained significant attention as non-viral gene delivery systems. These versatile macromolecules can form complexes with nucleic acids, protecting them from degradation and facilitating their delivery into cells. Polymers like dendrimers, often additionally treated with polyethylene glycol, offer advantages such as high transfection efficiency and the ability to carry different types of nucleic acids, such as plasmid DNA and mRNA.
- Peptide-based non-viral vectors
Peptides have also emerged as potential non-viral gene delivery vehicles. They possess properties such as high cellular uptake, endosomal escape, and low immunogenicity. Peptide-based vectors can be designed to interact with specific receptors on the cell membrane, facilitating targeted gene delivery. These peptides can be derived from natural sources or synthesized to possess desired characteristics for efficient gene transfer.
- Lipid-based non-viral vectors
An important non-viral vector type are lipid nanoparticles. Thanks to lipid bilayers, LNPs are able to encapsulate genetic material or drug substances. And by modifications on their surface, LNPs can be programmed to target specific cell types.1
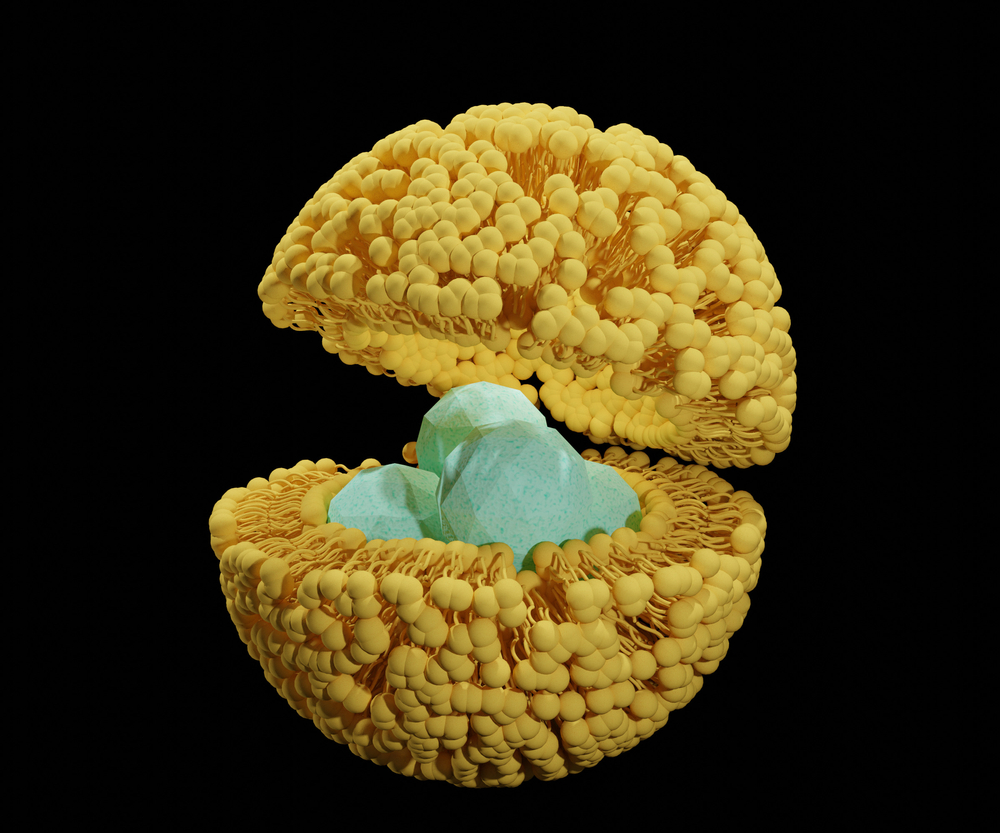
Mechanisms of non-viral gene delivery
Non-viral vectors utilize various mechanisms for cellular uptake, including receptor-mediated endocytosis, direct penetration, and macropinocytosis. Polymers, lipids and peptides interact with the cell membrane, facilitating their internalization into the target cells. This cellular uptake is crucial for the subsequent nucleic acid delivery into the cytoplasm.
Once inside the cells, non-viral vectors need to overcome intracellular barriers, such as endosomal entrapment, to ensure the efficient release of nucleic acids into the cytoplasm (i.e. intracellular delivery). They utilize endosomal escape mechanisms to avoid degradation and enable the transgene to reach the nucleus for transcription and subsequent gene expression.
Lipid-based nanoparticles (LNPs)
Lipid-based nanoparticles (LNPs) have emerged as versatile and effective non-viral gene delivery vectors, offering unique advantages in terms of efficiency, safety, and versatility. LNPs are composed of lipid bilayers and can encapsulate nucleic acids, including mRNA, DNA, and oligonucleotides, making them suitable for various biomedical applications.
Structure and composition of LNPs
LNPs are composed of lipids that self-assemble into nanoparticles with a lipid bilayer structure. The lipid components used in LNPs play a crucial role in determining their stability, delivery efficiency, and targeting capabilities.
- Liposomes – Liposomes are a type of lipid-based nanoparticle used as a delivery vehicle for nucleic acids. These vesicles consist of phospholipid bilayers that can encapsulate nucleic acids in their aqueous core. Liposomes can be modified by incorporating ligands or targeting moieties on their surface to enhance their specificity for particular cell types or tissues.
- Cationic lipids – Cationic lipids are essential components of LNPs and are responsible for interacting with the negatively charged nucleic acids and promoting their condensation into nanoparticles. The positively charged nature of cationic lipids enables their binding to the negatively charged nucleic acids and facilitates cellular uptake and endosomal escape.
Mechanism of action in gene delivery
LNPs utilize various mechanisms for cellular uptake, including receptor-mediated endocytosis and direct fusion with the cell membrane. The positively charged surface of LNPs, facilitated by cationic lipids and polymers, allows them to interact with negatively charged cell membranes and promote their internalization.
Once inside the cells, LNPs need to escape from the endosomal compartments to prevent degradation and ensure efficient delivery of nucleic acids into the cytoplasm. The pH-sensitive properties of certain lipids in LNPs enable their destabilization in the acidic environment of endosomes, leading to endosomal escape and subsequent release of the encapsulated nucleic acids.
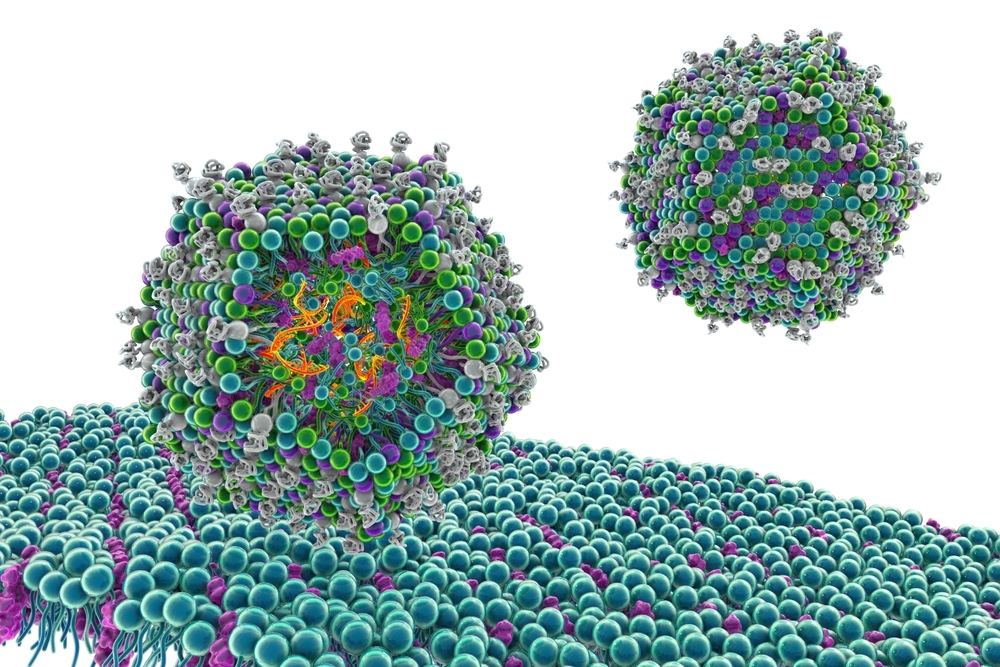
Advantages of LNPs
LNPs offer several advantages as gene delivery systems. They have high encapsulation efficiency, allowing for the efficient delivery of nucleic acids to target cells. LNPs also have the potential for tissue-specific targeting and enhanced cellular uptake through the incorporation of ligands or targeting moieties. Furthermore, LNPs have low toxicity compared to viral vectors and can be easily modified for specific applications.
Applications of LNPs in gene therapy
Lipid-based nanoparticles (LNPs) have gained significant attention in the field of gene therapy due to their potential for safe and efficient delivery of nucleic acids. LNPs offer a versatile platform for various therapeutic applications, including gene silencing, protein expression, and targeted drug delivery.
LNPs have been employed in various gene therapy approaches, such as the delivery of small interfering RNA (siRNA delivery) for gene silencing, antisense oligonucleotides for targeted inhibition of gene expression, and CRISPR components for precise genome editing. These approaches hold tremendous potential for treating a wide range of genetic diseases.
Lipid nanoparticles are being studied as an alternative approach to mobilization techniques of stem cells. By the means of LNPs, an in vivo delivery is hoped to ease the process of stem cell transplantation.
Additionally, LNPs offer a promising platform for targeted drug delivery. By encapsulating therapeutic agents within LNPs, drugs can be specifically delivered to target cells or tissues, minimizing off-target effects and improving therapeutic outcomes. This approach has potential applications in cancer therapy, where LNPs can deliver chemotherapy drugs directly to tumor cells, reducing systemic toxicity.
LNPs can also be utilized for the modification of immune cells, such as T-cells, to enhance their therapeutic potential in cancer immunotherapy. Through the delivery of genetic material using LNPs, T-cells can be engineered to express chimeric antigen receptors (CARs) or other immune-stimulating molecules, enabling targeted recognition and killing of cancer cells.2
Comparison with viral vectors
Lipid-based nanoparticles (LNPs) have emerged as a promising alternative to viral vectors in the field of gene therapy due to their unique characteristics and advantages. In this chapter, we will compare LNPs with viral vectors, specifically adeno-associated viruses (AAVs) and adenoviruses (AdVs), highlighting the key differences and benefits of using LNPs as gene delivery systems.
Safety considerations
LNPs are composed of biodegradable and biocompatible materials, reducing the risk of immunogenicity and adverse reactions. Unlike viral vectors such as AAVs or AdVs, LNPs do not possess the ability to replicate. Moreover, LNPs can be engineered to evade immune recognition and clearance, enhancing their stability and reducing the risk of immune responses.
Certain viral vectors, such as AAV vectors, have gained significant attention in clinical trials and have been approved for gene therapy treatments due to their favorable safety profiles and long-term gene expression.
Efficiency and transfection capacity
Viral vectors have traditionally demonstrated a high transfection efficiency due to their evolved mechanisms for cellular entry and nuclear delivery of genetic material. However, LNPs have made significant advancements in recent years, achieving comparable transfection efficiency levels, especially in specific cell lines and target tissues.
The optimization of LNPs' formulation, including the use of targeting ligands and modifications, has contributed to enhanced transfection capabilities.
Scalability and manufacturing
The production of viral vectors, particularly AAVs, can be challenging and time-consuming due to the need for complex manufacturing processes and the dependence on packaging cell lines. Thus, the large-scale production of viral vectors for clinical applications may pose practical limitations.
LNPs offer scalability and simplified manufacturing processes, making them amenable to large-scale production. The formulation of LNPs can be standardized and easily reproduced, ensuring consistency and reliability in gene delivery applications. Additionally, high levels of consistency, customization and scalability can be achieved.
Non-viral vectors offer more flexibility in terms of vector design and modifications. They can be engineered with specific targeting ligands, encapsulation materials, or surface modifications to enhance their specificity, stability, and cellular uptake.
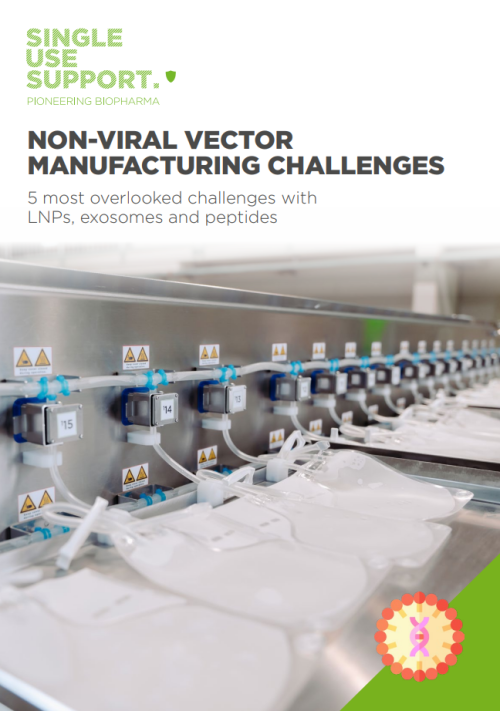
Download Guide
Download: Non-viral vector manufacturing - 5 overlooked challenges
Navigate the most overlooked challenges in non-viral vector manufacturing. Our guide covers topics like degradation and instability of LNP during freezing, scalability concerns in exosome manufacturing, homogenization of plasmid DNA in liquid transfer and much more.
Challenges with non-viral vectors in gene therapy
Gene therapy using non-viral vectors, including lipid-based nanoparticles (LNPs), has shown tremendous potential in overcoming the limitations of viral vectors. But despite recent advances, several challenges still need to be addressed for the widespread adoption and success of non-viral gene therapy approaches.
One of the main challenges associated with non-viral vectors is cytotoxicity and immunogenicity. Certain components used in the formulation of non-viral vectors, such as cationic polymers like polyethylenimine (PEI) and amino acids like lysine, may induce cellular toxicity and immune responses. Overcoming these challenges requires the development of safer and more biocompatible materials for gene carriers.
When a high level of gene delivery, a long-term gene expression or a large gene payload is required, viral vectors, such as lentiviral vectors and AAV, are still often used. And while LNPs have made significant progress in transfection efficiency, further improvements and clinical trials are still needed. Optimization of LNP formulations, including the use of targeting ligands, biomaterials like chitosan, and modifications to enhance cellular uptake and endosomal escape, can contribute to improved transfection efficiency and stability.
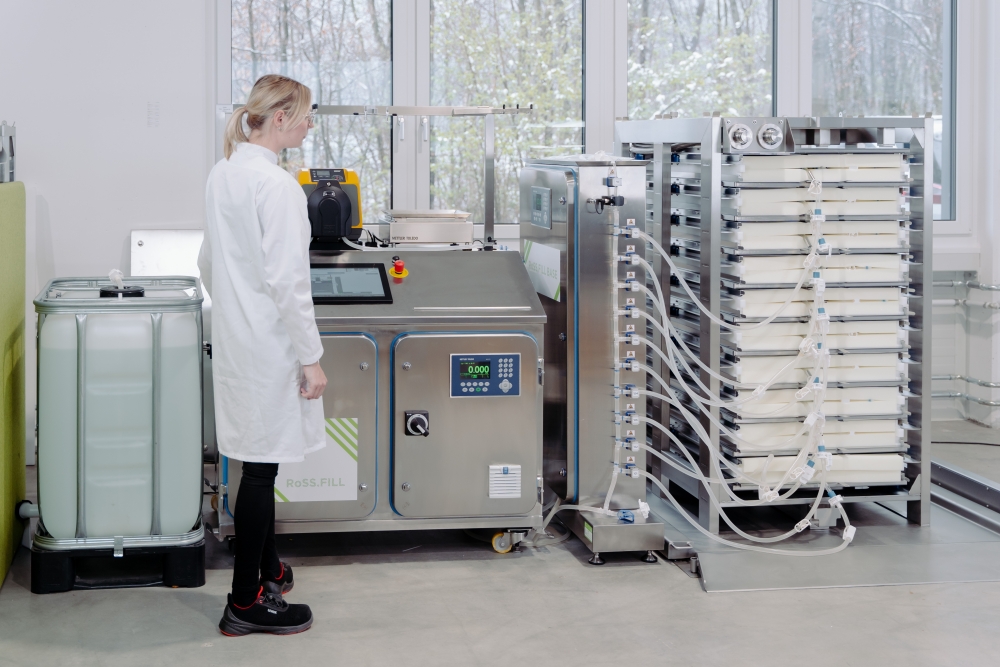
Boosting non-viral vector development with single-use technologies
Manufacturing scalability and cost-effectiveness are vital considerations for the commercialization of non-viral gene therapy. Traditional manufacturing processes involving complex steps, reagents, and multiple purification stages can be time-consuming and expensive. Furthermore, specialized processes like freezing LNPs demand for dedicated equipment, while processes like filling and aliquoting LNPs bring risks of product loss and cross-contamination.
Single-use technologies by Single Use Support offer a solution by providing a more streamlined and cost-effective manufacturing approach. Disposable consumables, single-use systems, and modular processes can reduce manufacturing complexity, increase flexibility, and improve overall efficiency. This is how single-use technologies can contribute to the huge progress that is being made in the field of non-viral vectors.
- Application of Non-Viral Vectors in Drug Delivery and Gene Therapy, http://dx.doi.org/10.3390/polym13193307, Published 2021-09-29
- Lipid nanoparticles produce chimeric antigen receptor T cells with interleukin-6 knockdown in vivo, http://dx.doi.org/10.1016/j.jconrel.2022.08.033, Published 2022-08-25