Viral vectors in gene therapy – the function, benefits and risks
Table of contents
ShowGene therapy holds the promise of revolutionizing medical treatments by harnessing the power of viral vectors to deliver therapeutic genes into the genome of patients. This innovative approach has the potential to address a myriad of genetic disorders and other diseases at their root.
However, as with any medical advancement, it comes with its own set of challenges – from an unwanted immune response and ensuring the safe and efficient delivery of proteins encoded by therapeutic genes to the manufacturing process of viral vectors.
In this blog post, we will illustrate these facets of viral vectors in gene therapy in detail.
How do viral vectors work?
Viral vectors work as carriers for genetic material, targeting specific cells. After entering these cells, they unleash their cargo into the cells’ genome in order to be replicated in vivo.
In the following sections, we will take a closer look at how human gene therapy vectors work.
Selecting the right target cells
The effectiveness of viral vectors hinges on their ability to selectively target specific cell types. Ex vivo, scientists and researchers carefully design these vectors to home in on the desired cell lines or cell types within the body, ensuring that the therapeutic payload reaches the appropriate destination. This specificity minimizes potential off-target effects and enhances the safety and precision of gene therapy.
One of the challenges in gene therapy using viral vectors is the potential for the host immune system to recognize the viral components and mount an immune response. The capsid proteins and viral genes can act as antigens, triggering an immune reaction. This immune response can potentially limit the effectiveness of the therapy or lead to adverse effects.
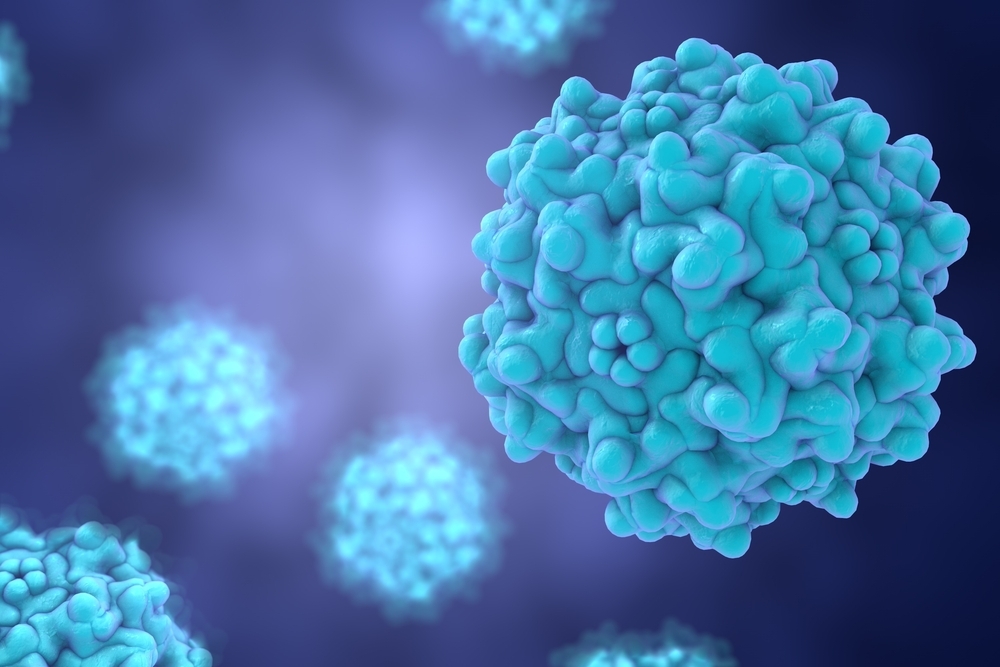
Navigating the cellular machinery
Transduction begins with the attachment of the viral capsid – the protective protein coat – to specific receptors on the surface of the target cell. This interaction triggers the entry of the viral vector into the host cell – be it a neuron or a liver cell. Once inside, the vector carries its cargo, which may include genetic material, therapeutic transgenes, or even whole genes, into the host cell's genome.
This journey may involve crossing various cellular barriers, such as the nuclear envelope, and accessing the host cell's chromosome, where it can integrate the therapeutic genetic material. The integration of the therapeutic genes is a critical step, as it allows for stable, long-term transgene expression.
Ensuring gene expression
The next critical step is the activation of the transgenes and the subsequent achievement of sufficient gene expression levels within the host cell. This complex process, often facilitated by specialized promoters, involves the transcription of the introduced genetic material into RNA, followed by the translation of RNA into functional proteins. These proteins are responsible for carrying out the therapeutic functions intended by the gene therapy.
Final outcome: Transduced cells with therapeutic potential
Ultimately, the successful journey of viral vectors within the host cell results in the transformation of the target cells into transduced cells capable of producing the therapeutic proteins or addressing the genetic deficiency. This transformation holds the key to the potential success of gene therapy, offering hope for patients with various genetic disorders.
Types of viral vectors in gene therapy
Some of the most widely used types of viral vectors are based on the adenovirus, delivering genes efficiently to a variety of cell types. AAV vectors (adeno-associated viral vectors) are a popular choice as well, known for their safety and ability to sustain long-term gene expression. Retrovirus vectors and lentivirus vectors are adept at integrating genes into the host genome, making them invaluable for diseases such as HIV (human immunodeficiency virus) research and gene therapy.
- Adenovirus vectors offer several advantages, including their capacity to deliver genes to both dividing and non-dividing cells. Adenovirus vectors come in various serotypes, allowing researchers to fine-tune their vectors for specific applications.
- AAV vectors, on the other hand, are favored for their low immunogenicity and ability to establish long-term, in vivo gene therapy. Adeno-associated viruses have become a staple in gene therapy research, particularly in the development of treatments for genetic disorders that necessitate continuous therapeutic gene expression.
- Retroviral vectors and lentiviral vectors are recognized for their unique ability to integrate the therapeutic gene into the host genome, offering a potential cure for genetic diseases caused by single-gene mutations. Notably, lentiviral vectors have played a crucial role in advancing research related to HIV-1 and hold promise for future gene-editing applications.
While these viral vectors hold immense potential for gene therapy, it's essential to address safety concerns. Some vectors, like replication-competent adenoviral vectors, need rigorous monitoring to prevent unintended consequences as well as deletion of certain genes. Additionally, the choice between episomal and integrating vectors, like lentiviral vectors, depends on the specific needs of the therapy, highlighting the importance of careful vector selection.
Furthermore, advancements in vector design have led to the creation of novel variants that enhance safety and efficacy. These variants are poised to expand the horizons of gene therapy, offering new possibilities for treating a wide range of genetic disorders.
1
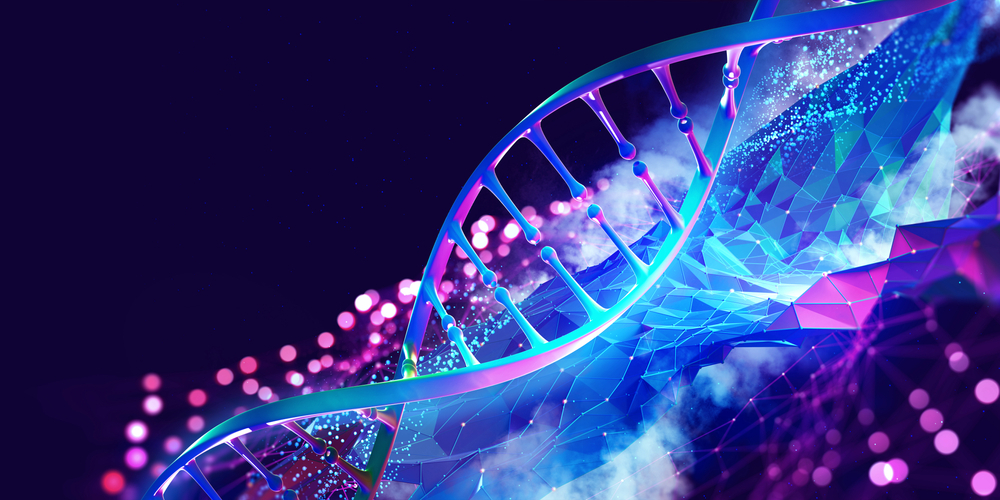
Viral vectors in gene therapy: Promising applications
Viral vectors are pivotal in the field of gene therapy, facilitating innovative treatments across a spectrum of medical applications. With innovative techniques like CRISPR, these vectors serve as potent vehicles for delivering therapeutic genes, shaping the future of healthcare.
Accordingly, several preclinical and clinical studies are being conducted to widen the already impressive field of application of viral vectors, as well as to improve in vitro manufacturing techniques. The importance is further highlighted by the fact that almost 70 % of gene therapy clinical trials conducted by 2017 involved viral vectors. Considering an overall number of nearly 3,000 trials, this number is all the more remarkable and explains the huge interest on biotechnology and regulatory authorities like the FDA in virus-based vector systems.2
In vivo gene therapy
In vivo gene therapy introduces viral vectors directly into the patient's body, offering lasting therapeutic benefits.
approach demonstrates promising results, e.g. applied on the nervous system or the liver.3
Viral vectors in cancer therapy
Viral vectors in cancer therapy deliver cancer vaccines, therapeutic genes or oncolytic viruses directly to cancer cells, triggering their destruction while sparing healthy tissues. These vectors can precisely target malignancies and also bolster the body's immune response against cancer, offering a precise and effective approach to treating various types of cancer.4
Gene therapy in immune disorders
Gene therapy applications extend to immune system disorders. By enhancing immune responses, these therapies offer new avenues for treating conditions like leukemia. Modified T cells engineered with viral vectors target cancer cells effectively.
Genetic disorders
Viral vectors hold immense potential in modifying genetic disorders such as cystic fibrosis or muscular dystrophy. They deliver functional genes to mitigate genetic mutations, paving the way for improved patient outcomes.5
Disadvantages of viral vector-based gene therapy
While viral vectors have revolutionized gene therapy, they are not without their challenges and drawbacks. It's essential to consider these disadvantages in the pursuit of safe and effective treatments:
- Immunogenicity – One significant concern is immunogenicity, where the body's immune system may recognize the viral vector as a pathogen and mount an immune response. This can limit the vector's effectiveness and lead to potential adverse effects.
- Insertional mutagenesis – In some cases, viral vectors can integrate into the host genome in unintended ways, causing insertional mutagenesis. This disruption of existing genes can have unpredictable consequences and poses a risk, especially in long-term treatments.
- Toxicity – Viral vectors themselves can be toxic to host cells, leading to cell damage or death. This toxicity can be particularly problematic in sensitive tissues or when high vector doses are required.
- Antibody formation – Antibodies against the viral vector can develop in response to treatment, reducing the vector's effectiveness upon subsequent administrations. This can complicate long-term therapies.
- Unwanted tumor growth in cancer gene therapy – In cancer gene therapy, where viral vectors are used to target and destroy cancer cells, there are concerns about off-target effects and the potential activation of oncogenes, which could promote tumor growth.
- Particularities of certain genetic disorders – Certain genetic disorders, such as hemophilia, pose unique challenges due to the need for precise delivery of therapeutic genes encoding complex proteins like clotting factors or glycoproteins.
- Neutralizing antibodies – Neutralizing antibodies can inhibit viral vectors' ability to deliver therapeutic genes, reducing the efficacy of treatment and complicating subsequent doses.
- X-linked disorders – In gene therapy for X-linked genetic disorders, effective gene transfer to a significant proportion of cells is often necessary, which can be challenging to achieve.
- Low ex-vivo viability – Viral vectors require special equipment and protocols in order to manufacture and store them ex vivo.
The benefits of single-use technologies in viral vector manufacturing
Single-use technologies have been accompanying viral vector manufacturing for years. These disposable systems reduce contamination risks, streamline processes, and enhance efficiency. They offer fast turnaround times between production runs and are easily scalable to meet varying demands, crucial for gene therapy applications.
While initial setup costs may be relatively high, single-use systems ultimately result in cost savings by reducing operational costs, eliminating cleaning, maintenance and validation associated with traditional equipment. Moreover, they contribute to a safer manufacturing environment, ensure consistent product quality, conserve resources, and simplify regulatory compliance.
Single Use Support has designed platform systems based on single-use technology that accompany the entire viral vector manufacturing process. While automated fluid management reduces risks associated with manual intervention, the plate-based freeze-thaw platform allows for fast and controlled freezing processes for viral vectors and related products. Contained by single-use bioprocess containers and covered by protective shields, viral vectors can be frozen to ultra-cold temperatures, ready for storing or shipping in ULT freezers.
To sum up, the platform systems provided by Single Use Support bring a set of benefits to viral vector production, including:
- reduced contamination risk
- fast turnaround times
- scalability
- cost-efficiency
- enhanced safety
- resource conservation
- modularit
- quality assurance
How are viral vectors used in CAR-T cell therapy?
Viral vectors play a crucial role in CAR-T cell therapy by delivering the genetic information needed to modify a patient's T cells. Initially, T cells are extracted from the patient's blood. Then, viral vectors, often lentiviral or retroviral vectors, are used to introduce genes encoding chimeric antigen receptors (CARs) into these T cells. These CARs enable T cells to recognize and target specific cancer cells when reinfused into the patient. This personalized approach enhances the immune system's ability to combat cancer.
What are typical non-viral vectors?
Non-viral vectors used in gene therapy include plasmids, liposomes, and nanoparticles. Plasmids are circular DNA molecules that can be introduced into cells to express therapeutic genes, but also to produce other recombinant enzymes, such as antibodies expressed by mammalian cells.
Liposomes and nanoparticles are lipid-based or synthetic carriers that can encapsulate and deliver genetic material into target cells without using viral components. While non-viral vectors may be less efficient at gene delivery than viral vectors, they offer advantages in terms of safety and reduced immune responses.
What are examples of viral vectors?
Examples of viral vectors commonly used in gene therapy and biotechnology applications include:
- Retroviral vectors
- Adenoviral vectors
- Adeno-associated viral vectors
- Herpes simplex virus vectors
- Viral Vector Systems for Gene Therapy: A Comprehensive Literature Review of Progress and Biosafety Challenges, http://dx.doi.org/10.1177/1535676019899502, Published 2020-01-24
- Viral Vectors in Gene Therapy, http://dx.doi.org/10.3390/diseases6020042, Published 2018-05-22
- , Published 1970-01-01
- Cancer gene therapy goes viral: viral vector platforms come of age, http://dx.doi.org/10.2478/raon-2022-0002, Published 2022-02-11
- , Published 1970-01-01